In the Early Spring of 2020
The mechanical engineering department of the University of Wisconsin–Madison (U.W.) was facing a stream of phone calls and e-mails from the local hospital. At first, there were shortages on face shields and masks, but soon it escalated into complex medical devices, such as ventilators and—most recently—power air-purifying respirators (PAPRs). Faculty associate Erick Oberstar and several other instructional staff and tenure-track professors in the U.W. College of Engineering felt the pressure—especially when they were the only ones allowed to stay on campus after all students had left.
Milwaukee, Wisconsin (USA)
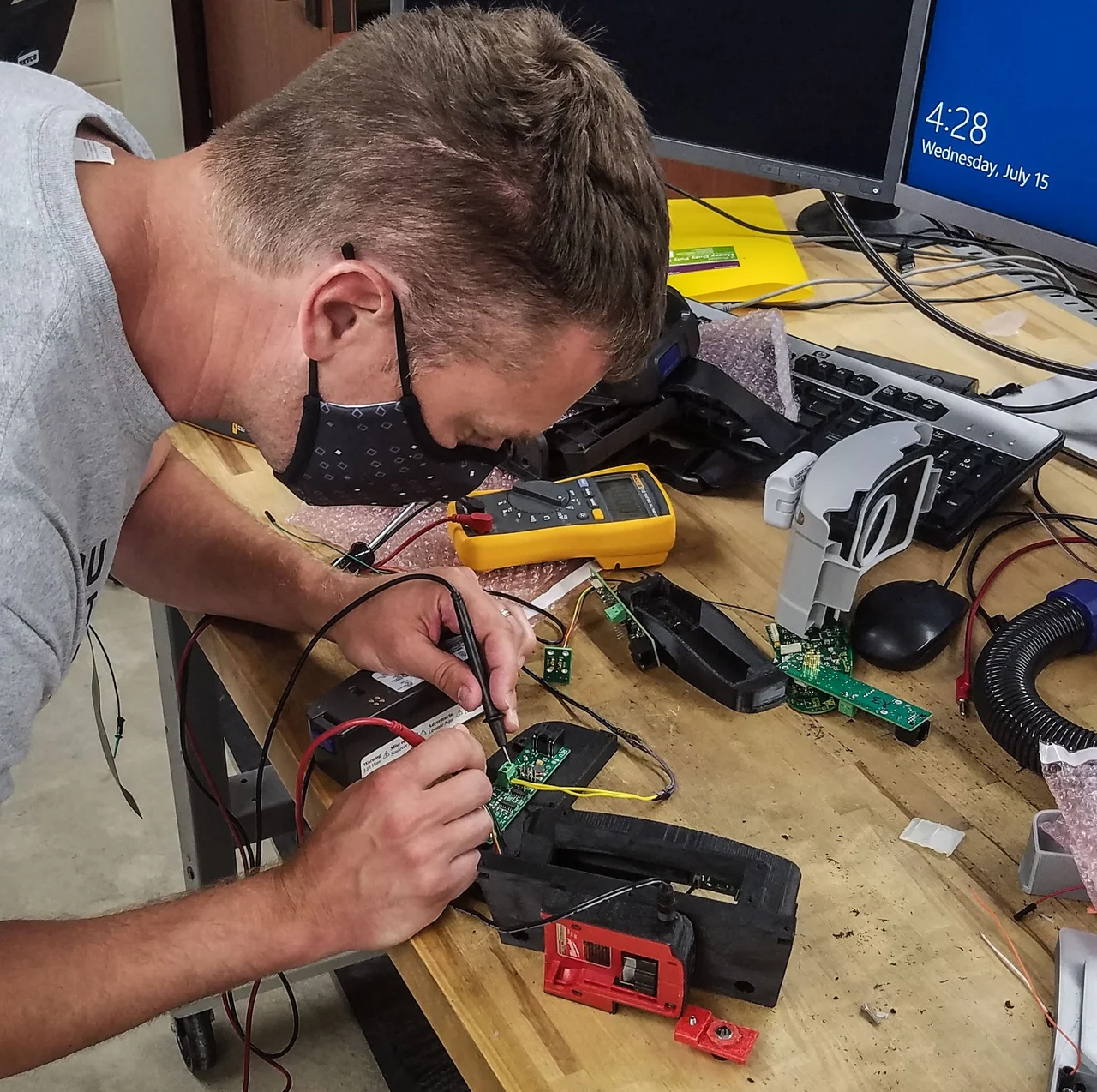
Faculty Associate Joe Krachey

and Assistant Professor Josh Roth at work on PAPR components in the University of Wisconsin Mechatronics Laboratory
What is a "PAPR"?
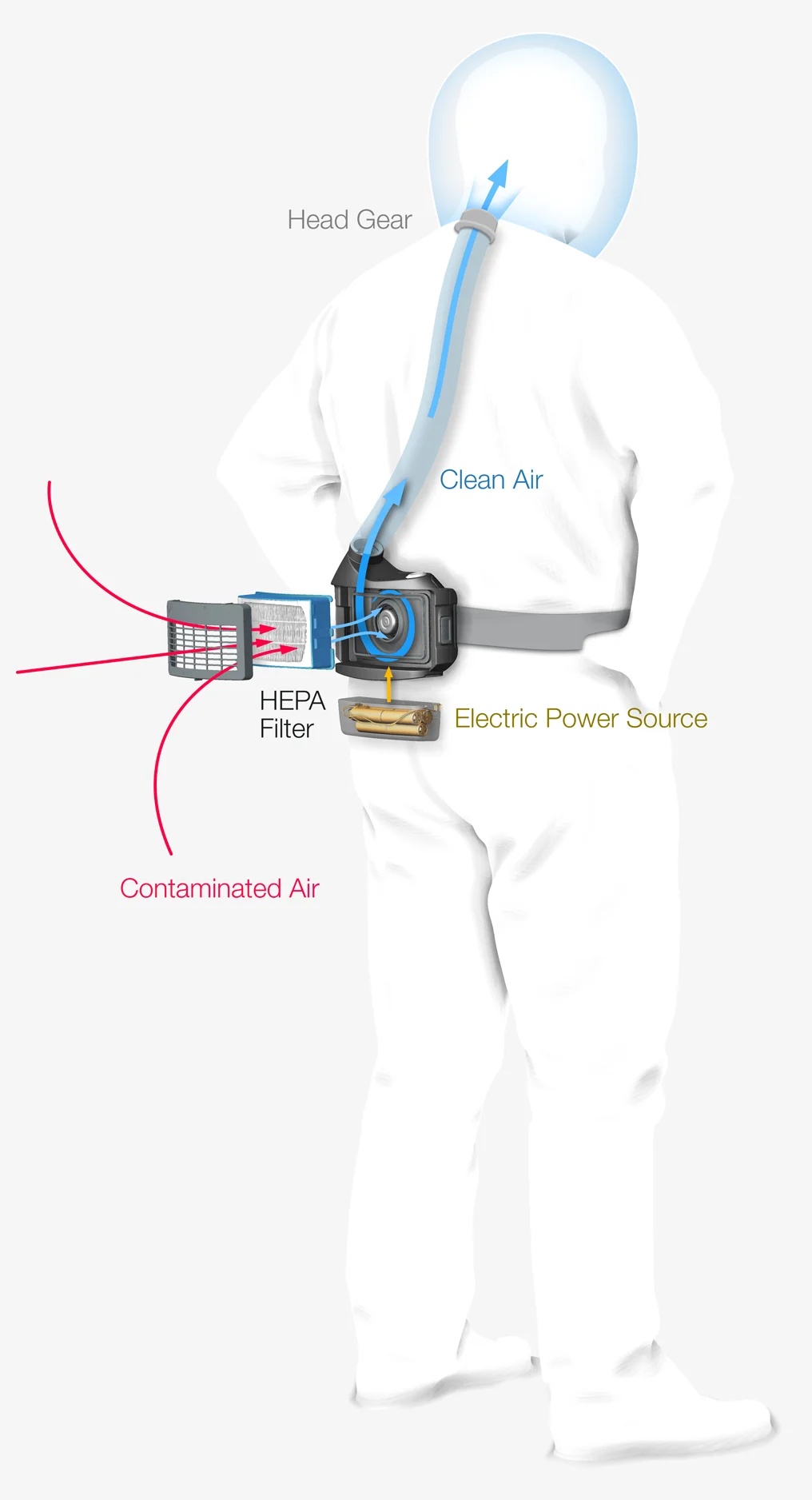
A power air-purifying respirator (PAPR) is used to safeguard workers against contaminated air.
It typically consists of headgear and a motor-driven fan that pulls contaminated air from the outside through a high-efficiency (HE) filter and delivers clean air to the user’s mouth and nose.
It is powered by a battery pack that runs the blower fans.
Because the user is encased in a sealed full-body suit, they are essentially working in a self-contained unit that constantly brings purified air in and keeps the virus out.
However, high efficiency comes at a price: the batteries that power the fans only run for several hours and require a multi-hour recharge, and the hospital did not have replacement batteries to switch them out for immediate turnaround. Even with enough battery power, the service life of the filters was running out—fast.
The mechanical engineering department was faced with a dilemma: PAPR devices had to be available for healthcare workers around the clock, but the battery packs that came with them could not keep up. An alternative power source needed to be used while the original battery packs were recharging, but each PAPR manufacturer had different battery shapes and filter geometries. It was then that they began looking elsewhere—what else was known for using rechargeable batteries and high-quality filters?
Power Tool Equipment

Where there is drilling, there is dust: a power tool battery in its natural habitat

Two industrial HEPA filters between 3D-printed adapter (blue) and fixation (red)
Power tool equipment also uses filters to keep out dust from concrete and other airborne particles resulting from construction work. The team tested industrial filters from a local company, and found that they met National Institute for Occupational Safety and Health (NIOSH) regulations for efficiency. Using these filters in modified PAPR devices could help fill the gaps in equipment when all other NIOSH-approved options have been exhausted.
Fortunately, both PAPR devices and power drills use high-efficiency particulate air (HEPA) filters, so it was a matter of rescaling industrial filters to meet NIOSH requirements for filter efficiency when it came to COVID-19 particles. After much deliberating and experimenting, the team calculated mating surfaces based on the cover of a two-part electric filter to create a geometry that would fit into the ductwork of the PAPR blower and provide enough surface area to be a viable filter replacement. This workaround would quickly allow them to meet NIOSH specifications and provide a field device that clinicians could use.
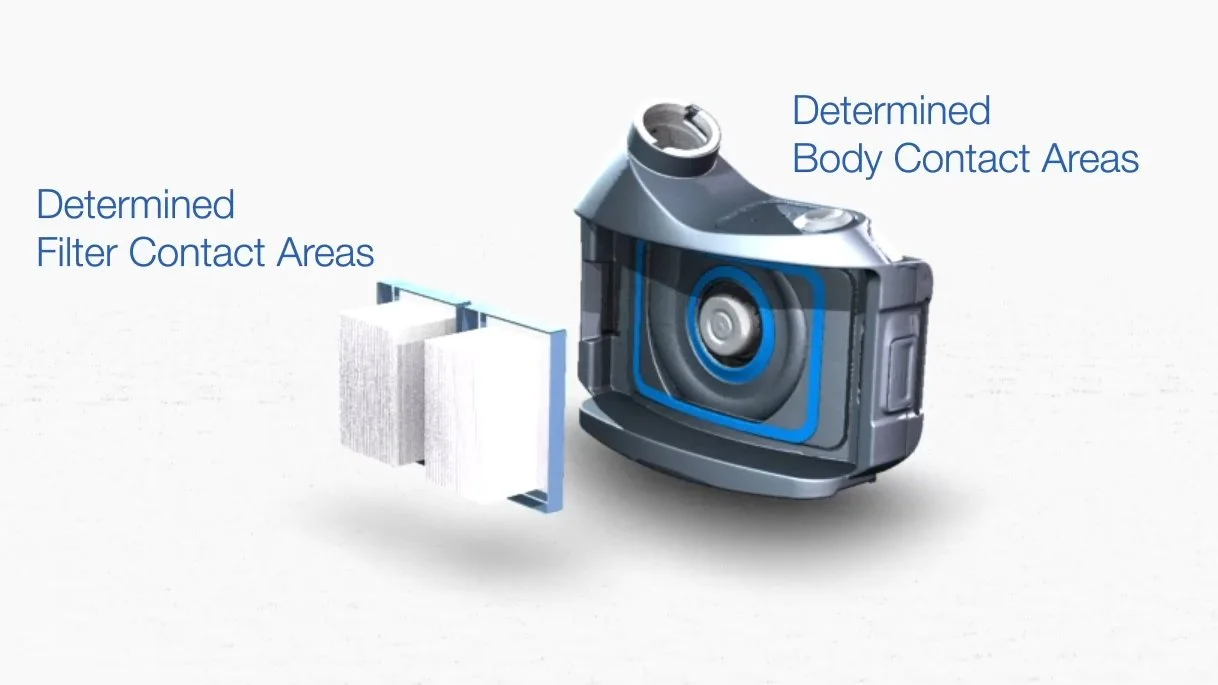
Pictured: the mid-stage prototype of the two-filter design. The calculated contact areas on the filter and the body will determine the shape of the component to be created.

Pictured: the resulting shape of the airtight component to be created (in the middle)
The challenge was achieving the exact dimensions necessary to produce critical, airtight filter components—in other words, achieving precision mating geometry of each part to ensure the filter would fit into the ductwork or that the battery pack would not fall out of the device. However, despite the high-quality CT scans of the device, a few unexpected problems arose. For one, contacting parts were made of the same material, and “airtight” meant just that—there were no gaps between them—which made the filter adapter a challenge to segment in VGSTUDIO MAX. For another, engineers found that brighter materials caused by connectors, soldering in the batteries, and dense glue distorted the gray values of the darker materials they wanted to reverse engineer.
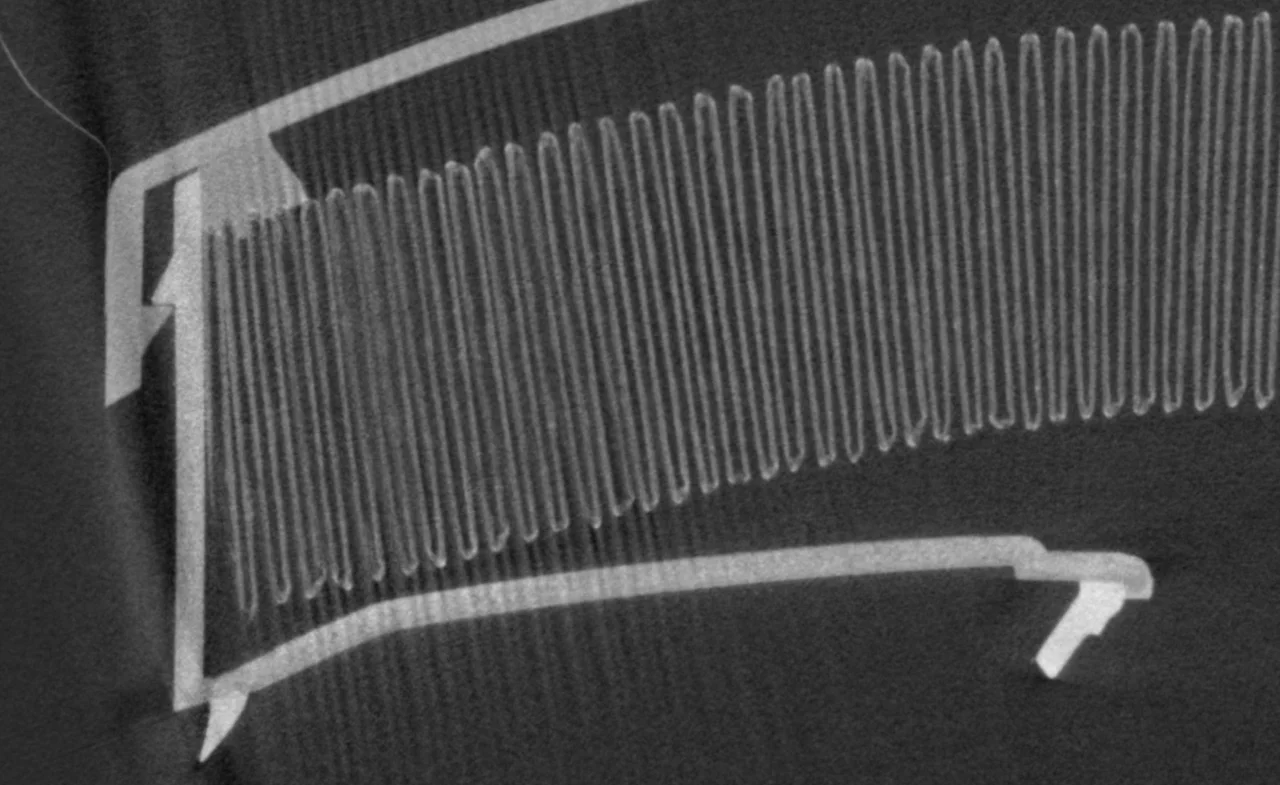
Segmentation challenges inside the original HEPA filter: the gray values are the same (plastic and glue), and there are no gaps

Segmentation challenge inside the original battery pack: brighter materials cause streak artifacts on plastic
The VG Solution
This is where the CT-data analysis and segmentation capabilities of Volume Graphics come into play. To understand the mating surfaces of a PAPR filter adapter, we need to determine the exact dimensions for each component: the rubber gasket, plastic resin, and filter material itself.

Exploded view of the original filter: a paper filter sealed in a two-part plastic housing

Another proposed filter-design: filter housing (red), gasket (green), cover (blue)
To achieve maximum resolution, we begin by scanning and importing several volumes and running a preliminary surface determination to get an idea of what the overall surface looks like. This also allows us to fit geometry elements on parts of interest to use for sequential registration, as the filter adapter offers canonical surfaces that could be used.
TIPS & TRICKS: Surface Determination
An advanced surface determination reconstructs the component geometry more closely by compensating deviations caused by external factors, like noise or other artifacts. Switch off contour healing to make it run faster; at this stage, we only want to align the parts. We can run a more refined surface determination later.

Preview of the surface determination
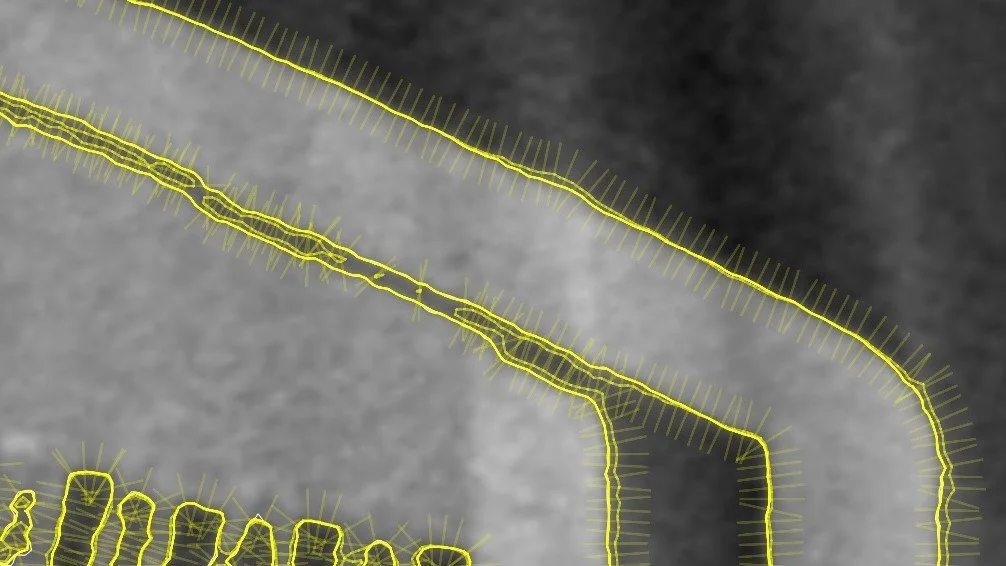
Close-up of the preview: The thicker yellow line represents the advanced surface determination. The thin line is the ISO50 line
TIPS & TRICKS: Sequential Registration
Sequential registration is the method of choice for matching geometry elements, as it allows you to align these elements in steps. By mapping matching geometry elements on two different objects, this method fixes degrees of freedom and places constraints on the remaining registration steps. The cool thing is, not all degrees of freedom need to be fixed—for example, a wheel can still rotate around one fixed axis. This comes in handy when you may not have geometry elements to fix all degrees of freedom.
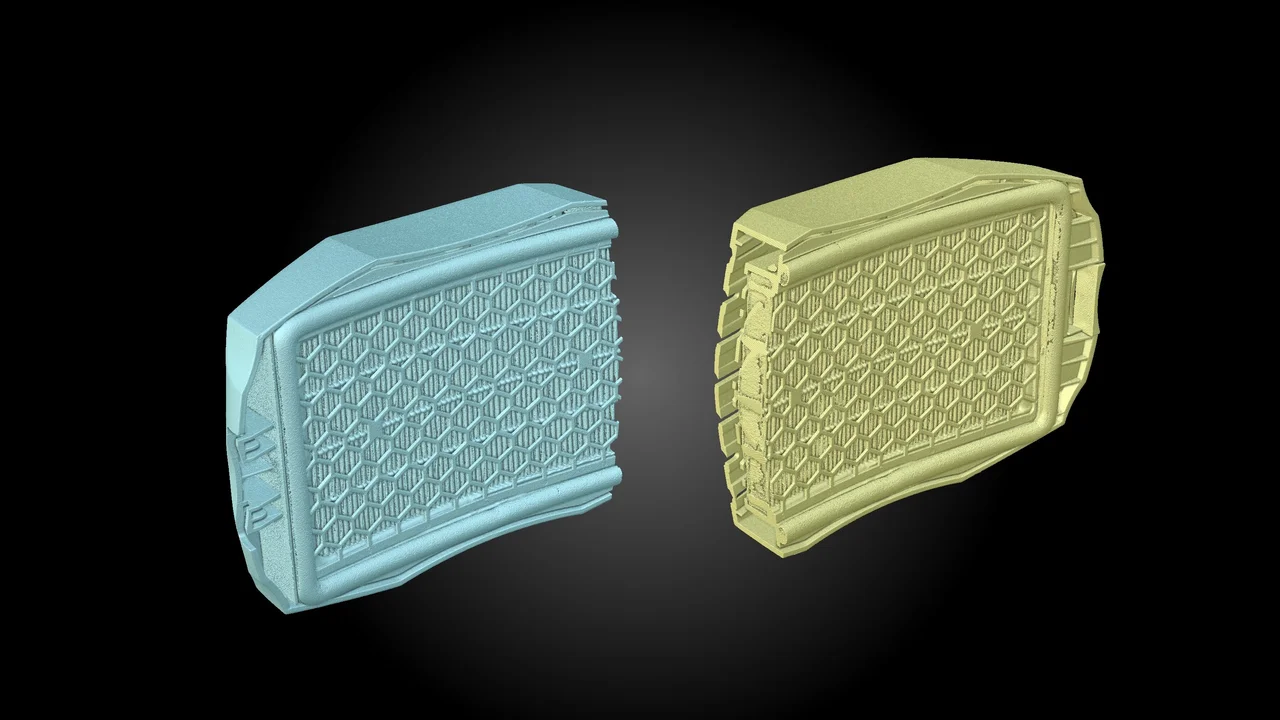
A HEPA Filter scanned in two stages to increase spatial resolution. Merging is to be done using the "Merge volumes" option

The two separate volumes aligned
So let's recap: We have several scans of parts in the Scene Tree, but we want to perform segmentation on all of them simultaneously. For that, we need to combine all scans into one volume, which leads us to the "Merge volumes" function of the software. As the name implies, this feature simply combines two volumes into one. For multiple scans, merging can be done iteratively. This becomes particularly useful when you have overlapping regions in both volumes, which is likely to occur when you scan two halves of a large object, and you want to determine what to do with them.
Did You Know? Merging Volumes
When combining two volumes, "Merge volumes" allows you to decide what to do with the gray values in the overlapping regions. It provides more options than multiple volume export.

The "Aligned/multiple volume export" dialog allows you to align more than two volumes at the same time as well as choose the mode of combination.

Now we have one all-encompassing scan of a filter adapter, but as you can see, it is not quite as clean as we want it to be. There is a lot of noise caused by differences in scanning—like the resolution of the detector—that affect scan quality. As we want to segment the components of the filter adapter based on gray value, we want to make sure the gray values are as smooth as possible.
In this case, we apply an adaptive Gauss feature, which examines and assigns importance to the surrounding voxels based on distance and position to the selected voxel. This is often preferred to the normal Gauss filter, which does not take these factors into consideration.

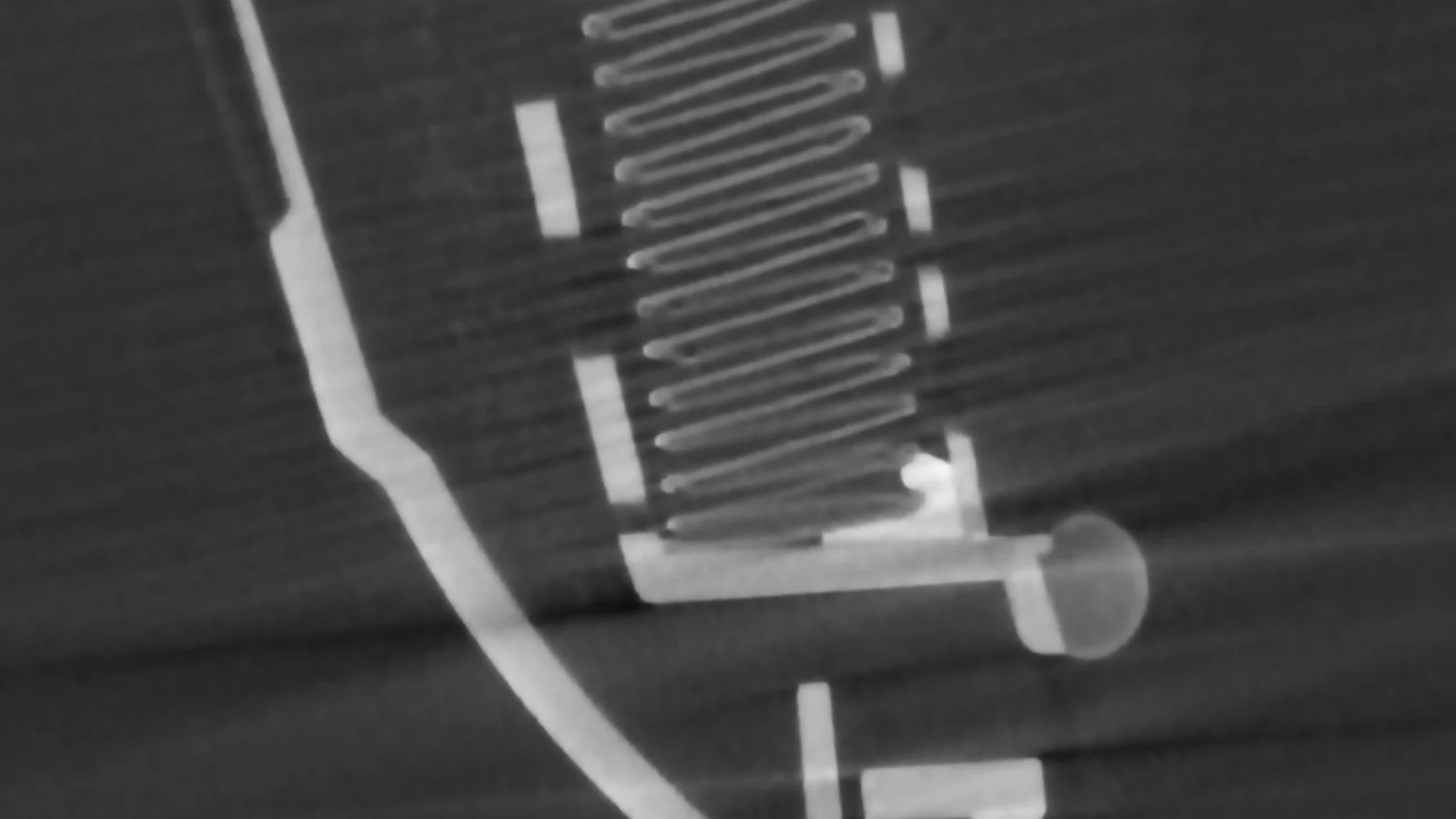
Adaptive Gauss OFF (left ) vs. ON (right)
This lays the groundwork for the main battle: segmentation. An advanced multi-material surface determination allows us to segment the filter adapter based on different gray values that correspond to different materials. This also helps us visualize the components in 3D and differentiate between the materials they are made of. By creating an ROI for the different surface components—i.e., materials—we have a good basis for a clean and accurate segmentation. First, we use the "Split ROI" function with a minimum volume to remove noise particles based on size. By using features like region growing, erode/dilate, and refinement, we can fill in regions that are not recognized as part of the segmented area and further optimize the segmentation.

Split ROI: the bigger the voxel count, the fewer the resulting ROIs
Reverse Engineering
By working with ROIs created from geometry elements, we can extract them along with the determined surface and use them as our starting contour. We can then convert the volumes to surface meshes. From there, we are able to derive and work with complex geometries and export them as a mesh to use for the reverse engineering process. Based on the exported mesh, we can design the missing parts and use 3D-printing to create rapid prototypes and polish the final shape.
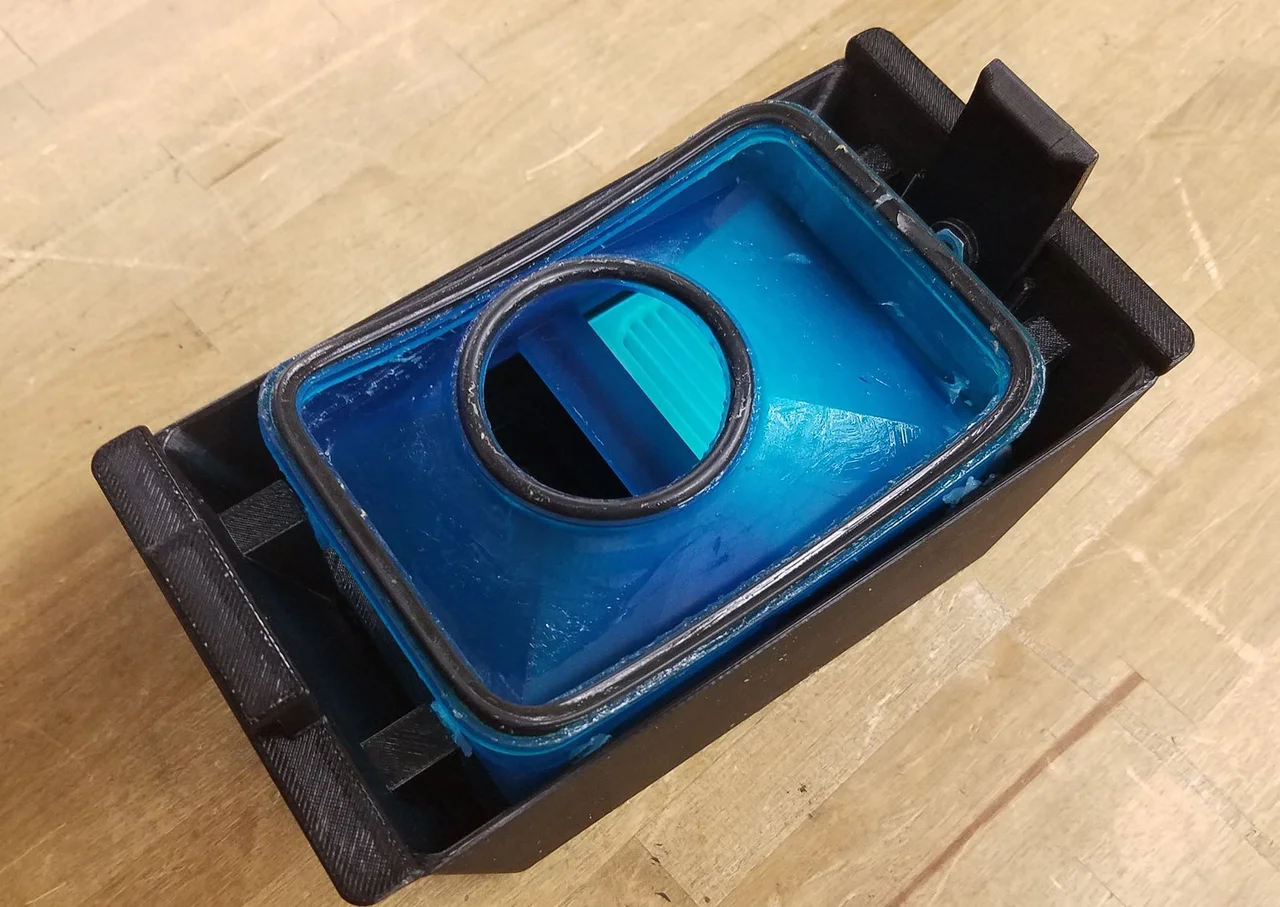
The blue component is a 3D printing of the redesigned prototype of the filter adapter. The cylindrical seal design was replaced with an O-ring, and the geometry of the rectangular seal was replicated using an inset gasket.
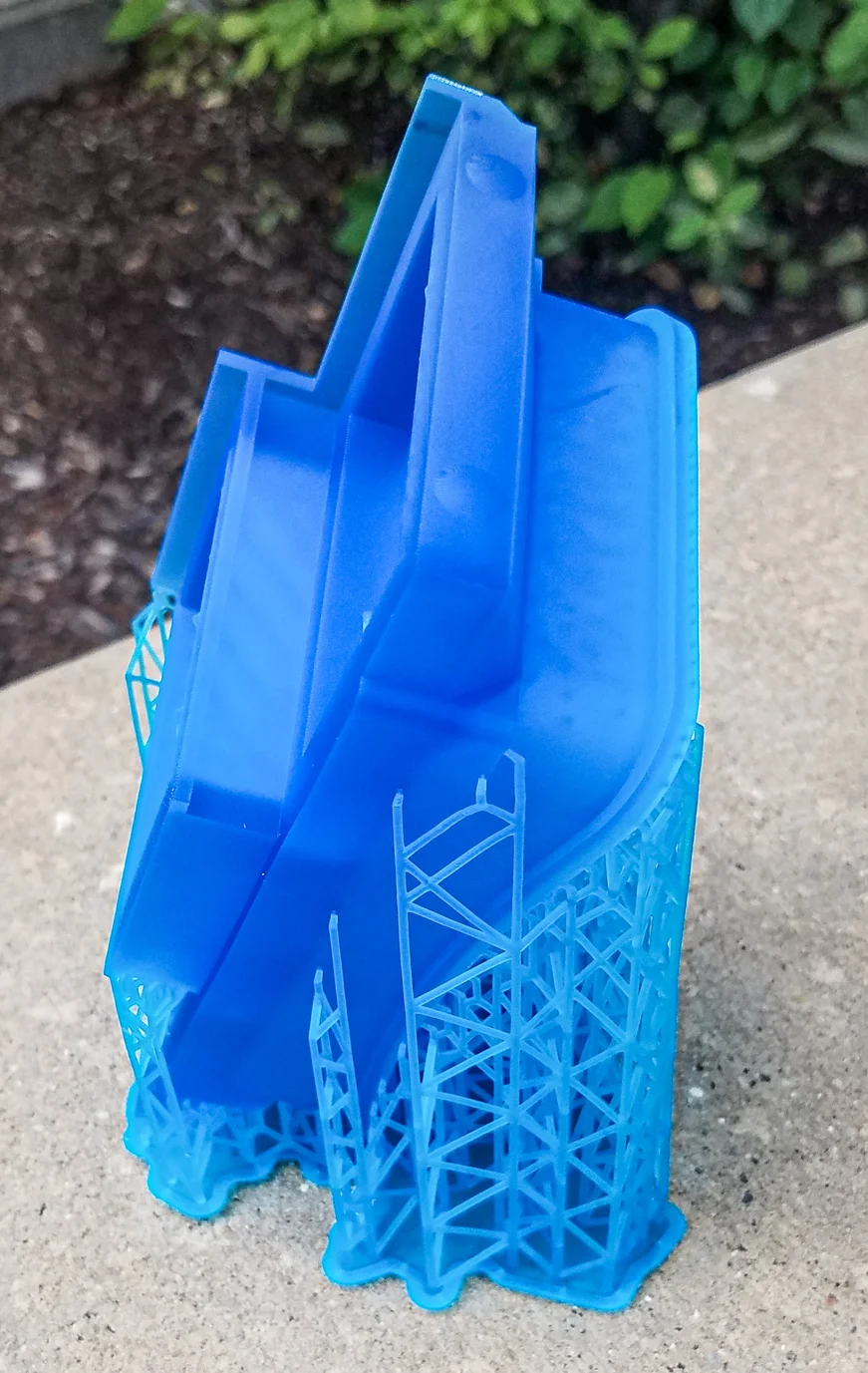
Stereolithography (SLA) 3D printing, which uses a liquid resin material to print materials, was used to create a model of the filter housing. Compared to other 3D printing methods, such as fused filament fabrication (FFF), it results in smoother, watertight. and pore-free structures.
This approach also helped solve the problem with the batteries. Engineers were able to secure over-the-counter power tool batteries that had adapters to adjust the voltage required by the PAPR fan. However, the adapters themselves did not match the PAPR device’s geometry. By scanning each type of adapter, we can segment the geometries of its plastic cover and design custom connectors that could be integrated into the PAPR assembly. This sounds simple in theory, but challenges come in all shapes and sizes—quite literally. For example, one of the greatest difficulties we encounter lies in replicating the snap-and-lock mechanism on the battery housing that prevents the batteries from falling out. This requires more attention to the contact zones of the part.

Image of snap-lock mechanism and contact zones
The innovative "convert-to-CAD" function of VGSTUDIO MAX also allowed us to generate CAD models of individual components—some of which were plastic, metal, or even glue—and extract critical mating surfaces for both filters and batteries. Engineers could then take that geometric data from the software back into their CAD system before moving on to production.

Power devices ready to be implemented!
EPILOGUE: DVC Function for Ideal Alignment
Now, we can use the digital volume correlation (DVC) function of VGSTUDIO MAX to align the various components. In essence, this functionality allows us to take two scans of the same part to see how an object changes—for example, due to temperature changes, stress, or simply time. The first step of the process requires aligning the scans based on the material, which gives us a clear advantage: a surface determination would no longer be needed to align the objects. In terms of our air filter adapter, which has such different components—for example, a rubber gasket, plastic housing, and metal—parts are much easier to align based on differing gray value information.

Both scans were aligned using DVC
The second step is comparing the volume data to identify any changes caused by external factors. In the case of our air filter adapter, this step is not necessary, because we would only use the DVC function to align our objects more effectively. However, this can be useful to inspect industry parts that undergo heat treatment, which often results in crack formation, deformation, and warping.


This is a comparison between the original device and the two-filter design. The two-filter design was a stepping stone to the three-filter design, which had sufficient filtering surface and battery power to meet NIOSH requirements.
Ready to learn more?
Users of VGSTUDIO MAX can find out more about segmentation, merging volumes, and the different registration methods in the tutorials included in the software.
Got a Story?
If you have a VG Story to tell, let us know! Contact our Storyteller Team at: storytellers@volumegraphics.com. We look forward to hearing from you.